Molecular Radiooncology
- Imaging and Radiooncology
- Clinical Cooperation Unit

Prof. Dr. Dr. Peter Huber
The goal of current molecular research in Radiation Oncology is to work towards a personalized medicine program, applying high-throughput methodologies in preclinical and translational research trials. Genetic key players in radioresistance and the determinants of recurrences after radiotherapy are being investigated to broaden the therapeutic window and improve clinical outcome in radiotherapy cancer patients.
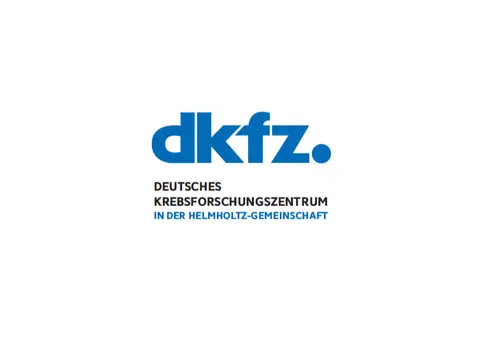
Our Research
The aim of our research is to improve radio-oncological tumor therapy. In particular, our work covers the field of radio-immuno-oncology with a focus on DNA damage and repair, tumor angiogenesis and fibrosis. We use low and high linear energy transfer (LET) radiation, including photons and charged particles (e.g. protons, carbon ions and others).
In addition, we are investigating rational combination therapies with a view to translational applications. These are designed to enhance the anti-tumor effect of radiotherapy and reduce side effects in normal tissue to extend the therapeutic window of radiotherapy.
Our preclinical experiments are carried out in cell cultures and animal models. We also initiate or participate in clinical trials, including reverse-translational programs to feed clinical data back into basic research.
Radio-Immuno-Oncology
Our research contributes to a better understanding of the effects of radiotherapy on the immune system in order to improve treatment success through combined radio- and immunotherapies. An important milestone was our finding that low-dose radiotherapy activates iNOS in macrophages, leading to normalization of blood vessels in the tumor and increased T-cell infiltration (Klug et al., Cancer Cell 2013). This work has laid the scientific foundation for low-dose radiation as a new clinically relevant therapeutic strategy. Higher doses of radiation, on the other hand, lead to interferon responses in macrophages, which are accompanied by the release of CXCL10. This and other chemokines released by the senescence-associated secretory phenotype (SASP) of irradiated tumor cells lead to further T-cell recruitment. In pancreatic cancer, the radiation-induced SASP chemokine CXCL8 also attracts highly cytotoxic CD56dim NK cells, which enhance the anti-tumor effect of radiotherapy (Walle et al., Science Advances 2022). In other tumor entities, CXCL8 otherwise correlates with poor prognoses in connection with tumor cell invasion, metastasis and angiogenesis due to the attraction of granulocytes. In pancreatic cancer, however, the positive effect through the recruitment of NK cells predominates. In xenotransplanted mice, the combination of radiotherapy and adoptive NK cell transfer improved tumor control compared to monotherapies. Our results point to a new class of combined immunotherapy and radiotherapy concepts with NK cells that could lead to a better therapeutic response in T-cell resistant tumors such as pancreatic cancer.
DNA Damage and Repair
DNA damage is the fundamental basis of the biological effects of radiation. Changes in DNA repair mechanisms contribute to therapy resistance in various cancers. We are investigating the basic molecular principles of radiation effects mediated by DNA damage and transfer our findings to therapeutic concepts in order to overcome resistance mechanisms. For example, we were able to visualize the internal structure of radiation-induced foci for the first time using superresolution microscopy. These are locally confined accumulations of repair proteins in the cell nucleus, which assemble or are activated at sites with double-strand breaks (DSBs) in the DNA. Only superresolution microscopy reveals what remains hidden by conventional microscopy: the foci of certain repair proteins consist of several small units, the ‘subfoci’ or ‘nanofoci’ (Lopez Perez et al., FASEB 2016). Their number correlates with the severity of the DNA damage and differs between irradiation with X-rays or carbon ions. The particle radiation leads to particularly difficult-to-repair DSB clusters, which are decisive for its increased effectiveness compared to X-rays (Lopez Perez et al., Radiother Oncol 2019). Various types of particle beams therefore have the potential to overcome the resistance mechanisms of tumors to conventional radiotherapy with X-rays (Hartfiel et al., Radiat Oncol 2019). However, their effects must also be considered in normal tissue cells, such as mesenchymal stem cells, both in the context of radiotherapy and in human spaceflight (Rühle et al., Cancer Lett 2022). In order to better understand the formation of DSBs and their consequences in the future, we are using the highly innovative sequencing method LAM-HTGTS in close cooperation with Dr. Pei-Chi Wei. This enables us to detect genome-wide DSBs caused by various clinically relevant types of radiation directly at the DNA sequence level and to map them with the highest accuracy.
Combination Therapies
Rational combination strategies of radiotherapy with targeted drugs are being researched primarily with regard to translational tumor therapy. In particular, we are investigating strategies that have the potential to simultaneously improve the anti-tumor effect of radiotherapy and mitigate side effects. For example, we have shown that Connective Tissue Growth Factor (CTGF) plays a key role in the development of radiation-induced pulmonary fibrosis. Inhibition of CTGF reduces pro-fibrotic immune effects of radiotherapy, which can reduce or even reverse pulmonary fibrosis (Bickelhaupt et al., JNCI 2017). On the cellular level, CTGF inhibition causes a reduction of pro-fibrotic polarized “M2” macrophages, mast cells, dendritic and mesenchymal cells, as well as a reduced release of pro-fibrotic cytokines and factors that cause extracellular matrix remodeling (Sternlicht et al., Respir Res 2018). Based on our data, clinical trials have been initiated, including for the treatment of idiopathic pulmonary fibrosis. At the same time, we have evidence that CTGF inhibition can improve the anti-tumor effect of radiotherapy, e.g. in an orthotopic glioblastoma mouse model.
Team
-
Prof. Dr. Dr. Peter Huber
Head
-
Dr. Ramon Lopez Perez
Researcher
-
Nicole Helker
Sekretary
Selected Publications
Walle T, Kraske JA, Liao B, Lenoir B, Timke C, von Bohlen Und Halbach E, Tran F, Griebel P, Albrecht D, Ahmed A, Suarez-Carmona M, Jiménez-Sánchez A, Beikert T, Tietz-Dahlfuß A, Menevse AN, Schmidt G, Brom M, Pahl JHW, Antonopoulos W, Miller M, Perez RL, Bestvater F, Giese NA, Beckhove P, Rosenstiel P, Jäger D, Strobel O, Pe'er D, Halama N, Debus J, Cerwenka A, Huber PE
Röhrich M, Leitz D, Glatting FM, Wefers AK, Weinheimer O, Flechsig P, Kahn N, Mall MA, Giesel FL, Kratochwil C, Huber PE, Deimling AV, Heußel CP, Kauczor HU, Kreuter M, Haberkorn U.
Lopez Perez R, Nicolay NH, Wolf JC, Frister M, Schmezer P, Weber KJ, Huber PE.
Bickelhaupt S, Erbel C, Timke C, Wirkner U, Dadrich U, Flechsig P, Tietz A, Pföhler J, Gross W, Peschke P, Katus HA, Gröne HJ, Nicolay NH, Debus J, Sternlicht MD, Seeley TW, Lipson KE, Huber PE
Osswald M, Jung E, Sahm F, Solecki G, Venkataramani V, Blaes J, Weil S, Horstmann H, Wiestler B, Syed M, Huang L, Ratliff M, Karimian Jazi K, Kurz FT, Schmenger T, Lemke D, Gömmel M, Pauli M, Liao Y, Häring P, Pusch S, Herl V, Steinhäuser C, Krunic D, Jarahian M, Miletic H, Berghoff AS, Griesbeck O, Kalamakis G, Garaschuk O, Preusser M, Weiss S, Liu H, Heiland S, Platten M, Huber PE, Kuner T, von Deimling A, Wick W, Winkler F
Klug F, Prakash H, Huber PE, Seibel T, Bender N, Halama N, Pfirschke C, Voss RH, Timke C, Umansky L, Klapproth K, Schäkel K, Garbi N, Jäger D, Weitz J, Schmitz-Winnenthal H, Hämmerling GJ, Beckhove P
Heidelberg Institute for Radiation Oncology (HIRO)
Our Clinical Cooperation Unit is member of the Heidelberg Institute for Radiation Oncology (HIRO).
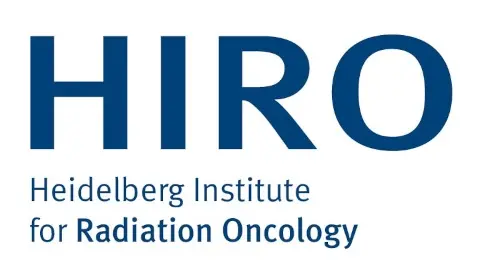
Get in touch with us
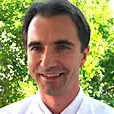