Research
The Teleman Lab is interested in understanding cell growth and tissue growth. We study two aspects of this problem: metabolism and signaling. To grow, cells and tissues need to synthesize biosynthetic building blocks to accumulate mass. This requires the activation of metabolic pathways that support anabolism and that fight Reactive Oxygen Species / oxidative stress. These metabolic pathways are regulated by signaling pathways such as the insulin, PI3K, TOR and Hippo/YAP signaling pathways.
Examples of projects are listed below:
ERC Consolidator Grant Project on Stearoylation
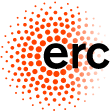
We just received an ERC Consolidator Grant to study protein stearoylation. We previously discovered that the lipid metabolite stearic acid can post-translationally modify proteins, and thereby affect cell signaling and animal physiology (see article). Interestingly, we recently discovered that our bodies are sensitive to the amount of stearic acid in our diets. The amount of stearic acid that we eat affects signaling pathways in our cells, thereby affecting our physiology. We are using a combination of techniques, from molecular and biochemical to organismal, to study how protein stearoylation affects our bodies. PhD and postdoc positions are available.
Regulation of TOR by amino acids
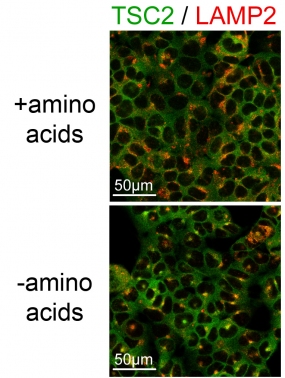
mTOR is a central regulator of cell growth. Highly conserved from yeast to humans, it is one of the most powerful anabolic signals in a cell. When mTOR is active, cells accumulate mass and grow, whereas when TOR is inactive, cells cannot grow. For this reason, mTOR is activated in almost all human cancers. mTOR promotes cell growth by inducing protein synthesis, as well as synthesis of other cellular building blocks such as lipids for the cell membrane. Because of its central role in regulating growth, mTOR activity is regulated by a large number of inputs including growth factor signaling (such as insulin), cellular stresses (such as hypoxia, or high AMP levels) as well as the availability of amino acids. The molecular mechanisms by which some of these inputs regulate mTOR, however, are still not understood. A few projects in the lab aim at identifying novel regulators of TOR, in particular relating to cellular amino acid sensing, and to understand their function both at the molecular level and at the physiological level (see papers). These projects are part of the local Collaborative Research Center SFB1036.
Regulation of mitochondrial morphology and function

Mitochondria are not only the "powerhouse of the cell" but also the site of important metabolic reactions such as the Krebs cycle, or fatty acid oxidation. Several projects in the lab are studying how mitochondrial morphology and function are regulated. In the past, we discovered that a lipid metabolite, C18:0, regulates mitochondria (see article). This identified a mechanistic link between a dietary metabolite (C18:0) and the functioning of mitochondria in the animal body. We are now doing a clinical study in collaboration with the group of Prof. Nawroth (Heidelberg University Hospital) to look at the effects of dietary C18:0 in people.
Regulation of tissue size during development

During animal development, tissues grow tremendously in size, growing roughly 1000-fold from the embryo to the adult. Each tissue then stops growing at a very precise size, which is determined by a combination of genetic and environmental factors. The precision of this growth controlling mechanism is illustrated by the fact that our limbs on the left and right sides of the body are almost identical in size. Surprisingly, the molecular mechanisms controlling tissue growth are not yet understood. Hence this represents a fundamental, basic, open question in developmental biology. Furthermore, it is of cancer relevance since the molecular pathways that control growth are linked to the re-initiation of proliferation observed in cancer.
In this context, we are studying signaling pathways that regulate tissue growth. These include:
(1) Insulin / PI3K / mTOR
(2) Hippo, Yorkie/YAP
(3) Dpp (TGF-beta in mammals)
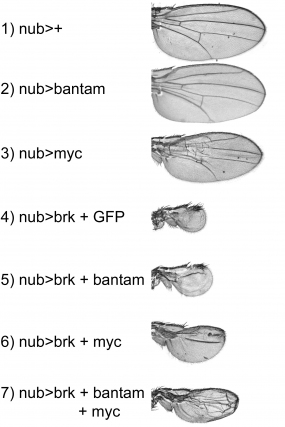
Since these signaling pathways are conserved from flies to humans, we use Drosophila as a model system for gene discovery and function elucidation. In fact, many components in these pathways were first discovered in the fly. The fly provides some major advantages, including a small genome, powerful genetic tools, and short reproductive cycle. This means, for instance, that knockout flies can be generated very quickly to assay gene function at the organismal level, at a speed that would not be possible with mammalian systems. We then translate our findings into mammalian systems.
One model system we work with is the fly wing, since it is a well-studied model system for tissue growth. The wing is specified as a group of 50 cells which then proliferate to yield a tissue with roughly 50,000 cells. When it reaches the correct final size, the cells in the wing stop proliferating. The underlying molecular mechanisms are not known. We are interested, for instance, in understanding how the Dpp morphogen gradient (see paper) controls growth (see paper). Furthermore, we aim to discover novel genes regulating this process.
Regulation of Translation
For information in our genome to be converted into phenotypes, the DNA needs to be transcribed to mRNA, and the mRNA translated into proteins. The proteins are then responsible for most phenotypes, including cancer. Recently, it has become clear that protein levels in a cell depend as strongly on regulation of mRNA translation as on transcription. In the past, much focus was placed on understanding transcriptional regulation and transcription factors. In comparison, regulation of translation is less well understood, in part due to past technical difficulties in measuring protein levels and translation rates. Recent technological advances such as ribosome footprinting and quantitative mass spectrometry now enable us to study translational regulation. For instance, we recently discovered that the oncogenes DENR and MCT-1 promote a process called translation reinitiation, to promote expression of genes required for proliferations (see article). Several projects in the lab focus on how translation is regulated, and how its dysregulation contributes to cancer.
Reactive carbonyl species and diabetes
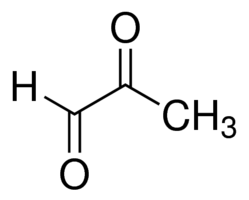
Some metabolites serve useful purposes. Some metabolites, such as glyoxal, methylglyoxal, or H2O2 are reactive and form adducts on proteins to disturb their function. Such reactive metabolites have dedicated cellular detoxification systems which degrade them. Elevated levels of metabolites such as methylglyoxal, which derive from glycolytic intermediates, have been linked to human diseases such as diabetes. Several projects in the lab study the detoxification of methylglyoxal, and the consequences of impaired detoxification for diabetes. These projects are part of the Collaborative Research Center SFB1118.
Aging
Metabolic homeostasis has been linked to aging in multiple ways. For instance, dietary restriction is one of the main lifestyle interventions known to delay aging. Exercise also delays aging and this occurs via modulation of cellular metabolism as well as signaling through nutrient-responsive pathways such as AMPK. Thirdly, nutrient supply regulates acetyl-CoA levels in cells, thereby affecting histone acetylation, which has been linked to aging. Finally, one of the main nutrient-sensitive kinases, mTORC1, is a potent modulator of aging in multiple animals including flies and mice. Indeed, a recent clinical study by Novartis showed that mTORC1 inhibition can partially revert one aspect of aging in humans - immunosenescence.
Since we have a focus on metabolic homeostasis, the biological processes that we study impact aging: mTOR signaling, mitochondrial function, and detoxification of reactive carbonyl species. Several projects in the lab analyze the impact of these processes on animal aging.