Research group Metabolism of Infection- and Inflammation-induced Cancer
Research theme…
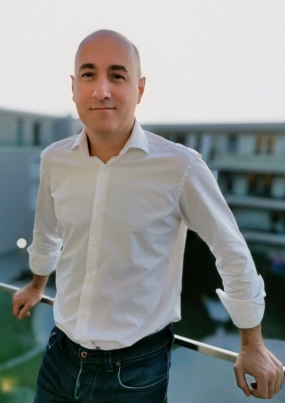
Dr. Bruno Galy, Ph.D.
© dkfz.de
Malignant tumor cells exhibit different metabolic requirements compared to most normal differentiated cells. Because such alterations can promote tumor initiation and metastatic progression, targeting the specific metabolic needs of cancer cells may be of therapeutic value. It is therefore of utmost importance to better understand the processes that lead to metabolic alterations in cancer. Our lab investigates the molecular bases that underlie metabolic reprogramming in cancer using iron metabolism and its (dys)regulation as a model system.
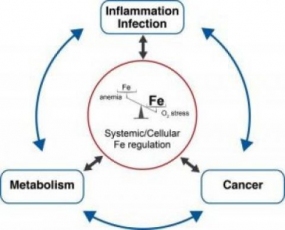
© dkfz.de
Both iron deficiency and excess are detrimental…
Iron is a co-factor required for numerous metabolic processes but toxic when present in excess, and imbalances of iron metabolism account for some of the most common diseases. Alterations in iron metabolism have recently emerged as a key metabolic “hallmark of cancer”. The metal helps cells to proliferate, and intrinsic alterations in cancer cells tend to enhance cellular iron availability while microenvironment cells seem programmed to fuel cancerous cells with iron. When present in excess, iron promotes the generation of free radicals, which cause DNA lesions and produce an inflammatory environment favouring tumor formation. On the other hand, iron is required for e.g. maintaining genome integrity; it also mediates ferroptotic cell death in response to specific anticancer compounds. The role of iron in cancer is thus complex and subtle changes in the iron balance in cancer versus surrounding cells can thus influence tumor initiation, progression, and treatment in multiple ways.
Importantly, iron plays a central role in host-pathgogen interactions. Indeed, microbes require iron to thrive and multiply, and the infected host mounts a innate response that aims at withholding the metal from the invader, a process termed “nutritional immunity”. Not surprisingly, viral infection as well as aseptic inflammatory stimuli also trigger major changes in iron metabolism, as illustrated by the hepatic iron loading that often accompanies e.g. hepatits C virus infection or non-alcoholic steatohepatitis. The study of iron metabolism thus offers an ideal context to better understand the crosstalk between metabolism, infection/inflammation and cancer.
How to achieve iron homeostasis…
In mammals, iron metabolism is balanced by two major regulatory systems:
- One controls systemic iron fluxes and relies on the liver hormone hepcidin and its receptor ferroportin (FPN) (Fig. 1).
- The other orchestrates cellular iron metabolism through iron-regulatory proteins (IRP)-1 and -2, two proteins that bind cis-regulatory iron-responsive elements (IRE) present in mRNAs encoding iron management proteins (Fig. 2).
Our lab uses state-of-the-art cellular and animal models to investigate the role(s) of these central iron regulatory networks in infection and inflammation-induced tumor formation. One focus is on liver cancer, which is frequently linked to infection with hepatitis B or hepatitis C virus and thus represents a paradigm of pathogen- and inflammation-induced carcinogenesis. We are also interested in investigating the role of iron in colorectal cancer, in which the microbiome plays an important role. In the long run we plan to exploit the tools and approaches developed for the study of iron metabolism to investigate other metabolic regulatory networks important for cancer.
Figure 1: Dietary iron is taken up by duodenal enterocytes, exported into the circulation via the iron exporter ferroportin (FPN), and loaded onto transferrin. Transferrin-bound iron is then distributed to tissues and is largely used for heme synthesis in red blood cells. Tissue macrophages recycle iron from effete erythrocytes and export it back into the circulation via FPN. The non-used iron is stored in hepatocytes. The hepatic hormone hepcidin lowers plasma iron levels by inhibiting the FPN iron exporter present at the surface of enterocytes and macrophages. Hepcidin synthesis is in turn regulated by plasma iron levels.
(click to enlarge the image)
Figure 2: In iron-deficient cells, both IRPs bind to the 5’untranslated region (UTR) IRE of the ferritin H- and L-chain mRNAs to inhibit their translation and thus decrease iron sequestration, while their binding to the 3’UTR IREs of the transferrin receptor 1 (TFR1) mRNA prevents its degradation and increases cellular iron uptake. In turn, iron repletion decreases IRE-binding via iron-sulfur cluster assembly on IRP1, causing its conversion to a cytosolic aconitase, and iron-targeted degradation of IRP2. Additional IRP-target mRNAs identified so far encode the FPN exporter, the DMT1 iron uptake molecule, as well as proteins involved in energy metabolism (ACO2), heme synthesis (ALAS2) and response to hypoxia (HIF2alpha).
(click to enlarge the image)
Current projects...
- Role of iron metabolism (dys)regulation in tunmorigenesis: “IRONing out” cancer metabolism.
- Fundamental roles of Iron Regulatory Proteins: Why is iron metabolism regulation so important for life?
- Define the regulatory scope of Iron Regulatory Proteins: how many IRP targets are present in the transcriptome?
- Establish an atlas of RNA-binding proteins active in tissues: How do cells shape their proteome?